Small molecule drug: past, present and future
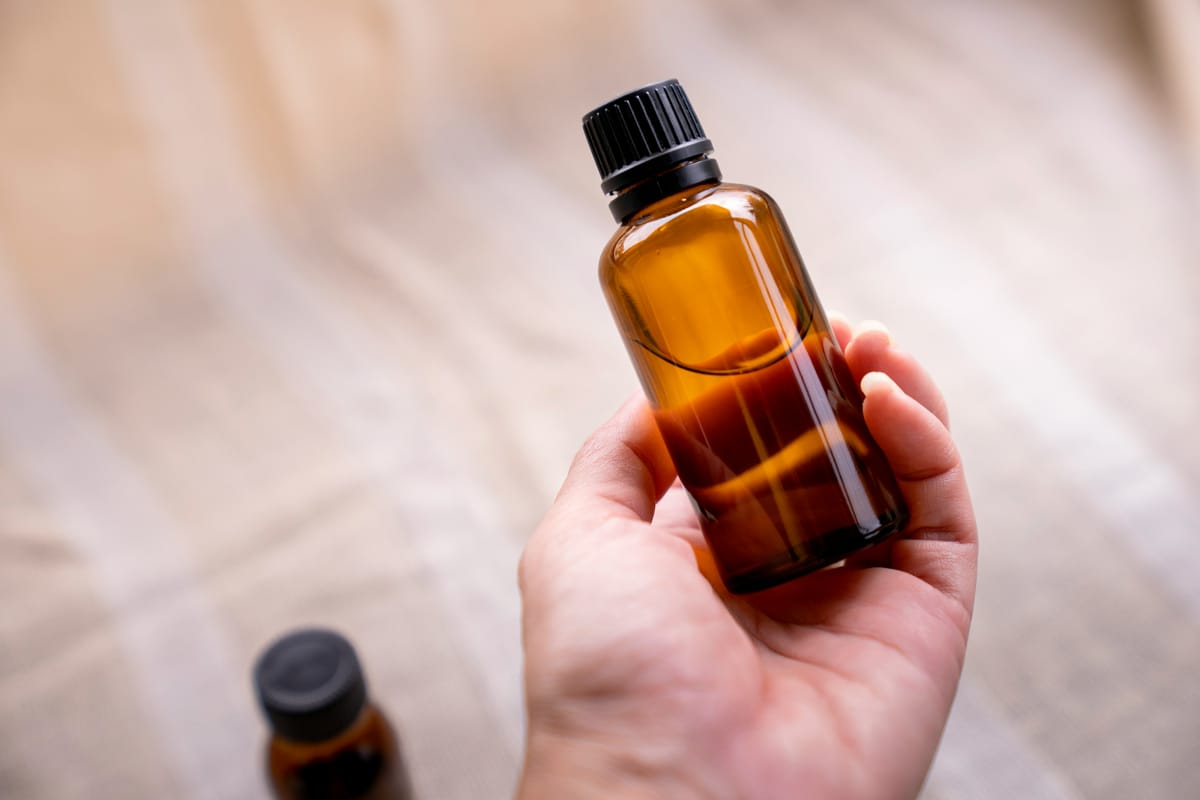
Traditional small-molecule drugs have been the dominant modality in drug research over the past century. Ever since the first synthetic drug, chloral hydrate,1 was discovered in 1869, small molecule drugs have played an instrumental role in progressing modern medicine and profoundly impacted the society. Apart from the well-known aspirin (one of the first synthetic prodrug for reducing inflammation and risks of cardiovascular diseases) and penicillin (serendipitous discovery that saved countless lives during WWII), some other prominent small-molecule drugs include Enovid (first hormonal oral contraceptive pill that gave women the power to control fertility and contributed to women’s emancipation), Retrovir (first combination anti-HIV drug that turned a deadly disease into a manageable chronic condition and established the use of small molecules for antiviral treatments that eventually led to effective medicines for COVID, Paxlovid, and HCV2), Xarelto (first direct factor Xa inhibitor for antithrombotic medication that combats cardiovascular diseases which have become the 21st century’s leading cause of death globally), and Vitrakvi (first tumor type-agnostic TRK kinase inhibitor that is effective in treating many types of cancers, different from the targeted cancer drugs like Novaldex and Gleevec).
Small molecules offer several key advantages over biologics. Their modular nature allows them to be decorated with different functionalities to target a diverse range of biological targets like proteins (enzymes, ion channels, receptors), oligonucleotides, lipids intracellularly and extracellularly. The flexibility of their structure allows for various modes of interaction with the targets. They demonstrate good permeability across biological barriers and oral availability and high metabolic stability to show high oral availability. The ease of chemical synthesis allows for tunability of their structure for optimization of the desired properties. They generally show low immunogenicity and minimal limitation in administration routes, leading to various formulation and delivery methods. Their long shelf-life stability and mature well-defined current good manufacturing practice (CGMP) makes their manufacturing, regulation, distribution and storage an ease and keeps the cost low.
The conventional modes of actions small molecules assume to elicit a therapeutic response include functioning as enzyme inhibitors like Gleevec, receptor agonist like Tirzepatide or antagonists like Tamoxifen, ion channel modulators like those targeting the cystic fibrosis transmembrane conductance regulator (CFTR) Kalydeco and Mytesi. Advancement in basic research has expanded our understanding of the cellular processes involving the therapeutic targets and inspired new chemical modalities and modes of actions of small molecule drugs, allowing drugging the previously undruggable targets.
For example, small molecule drugs can achieve targeted covalent inhibition (TCI) by covalently binding to the target site (Lumakras that covalently bind to the cysteine residue in the mutated KRAS proteins in solid tumors with G12C mutation). Small molecules can also act as potent protein-protein interaction (PPI) modulators (Selzentry for inhibiting chemokine receptor and viral envelope protein for HIV treatment) even though there are predominantly antibody-based PPI. Previously thought to contain no suitable binding sites for small molecules, RNAs have increasingly become the target of small molecule drugs due to their discrete secondary and tertiary structures (the first RNA-targeting small molecules, RSMs, Evrysdi acting as a splicing modifier on SMN2 mRNA to increase the functional SMN protein for treating spinal muscular atrophy was approved in 2020). Antibody-drug conjugates (ADCs) have gained increasing popularity recently (13 approved by FDA so far, 3 expected for approval in 2024-25) due to their specificity (from the highly selective antibodies) to direct the small molecule module to the right target site to exert its pharmacological activity. Examples include Kadcyla to treat HER2-positive metastatic breast cancer and the most recently approved ELAHERE to treat platinum-resistant ovarian cancer. Small molecules also enable targeted protein degradation by functioning as proteolysis targeting chimeras (PROTAC), lysosome-targeting chimeras (LYTAC), and molecular glue. PROTACs are bifunctional drug conjugates that contain two moieties that target a protein of interest (POI) and an E3 ligase to induce POI degradation respectively covalently linked to each other via a linker, while molecular glue is a monofunctional group that bind to an E3 ligase to increase its binding affinity with the POI. LYTACs target membrane and extracellular proteins. Therapeutic peptides have also demonstrated great potency and selectivity for inducing therapeutic response like semaglutide and tirzepatide. Small molecules play a vital part in facilitating gene and cell therapy. They ensure product quality via proper control of embryonic stem cell propagation, T cell expansion, and prevention of cryopreservation-induced cell death. In gene therapy, small molecules help to enhance vector internalization, improve transduction efficiency and block cellular antiviral pathways. Before being optimized for in vivo therapeutic effect, small molecules serve as chemical probes to understand the biology of the disease. They can be introduced to a disease model and studied for their ability to manipulated the target of disease, serving as a starting point for drug discovery that can be optimized later for in vivo efficacy. For example, vemurafenib started from a collection of probes for modulating melanoma cells and was later fine-tuned with additional features to work in vivo.
Today, small molecules are assuming a more diverse role in modern medicine and often possess multiple modalities to tackle complex diseases. With the advancement of new technologies like machine learning-assisted chemical space screening to predict hits and pharmacokinetic optimization. Free-energy perturbation simulations have benefited from the massive improvement in computing power to realize more accurate de novo designs. Retrosynthesis planning algorithms continue to improve to solve new synthetic problems and enlarge the small molecule drug chemical library. Big pharma companies have started to partner with AI computation providers to upgrade their drug discovery toolbox. The first three small molecules discovered with the aid of AI have hit the clinical phases (DSP-1181, EXS-21546, and DSP-0038)3. Combining with the ever-growing computational technologies and deepening knowledge to target or complex with biological entities, small molecules with their inherent advantages like infinite structural flexibility remain as a key tool in drugging the undruggable in future.
Reference
1. https://doi.org/10.1002/dta.301